Study
Reconstruction of severely resorbed alveolar ridges using bone grafting materials is essential for successful dental implant installation. bone grafts with three-dimensional stability in the form of blocks and shells are indicated for such extensive bone defects. Customization of bone blocks is another advantageous opportunity for the restoration of defects of greater complexity. In this regard, processed allogeneic bone grafts, among which are maxgraft® blocks, maxgraft® bonebuilder, and maxgraft® cortico, offer reliable solutions for a wide range of challenging situations.
The desire for implant-borne prosthetic restoration has become increasingly common among patients due to their favorable outcomes in terms of functionality, esthetics, and comfort.1 In order to achieve long-term success and guarantee optimal stability and esthetic outcomes, implants should be placed in the correct three-dimensional position in the dental arch with about 1.5-2 mm of bone around the implants.2 However, atrophy of the alveolar ridge following tooth loss or defects resulting from a periodontal disease or trauma compromise oral rehabilitation with implants. Hence, bone augmentation procedures using grafting materials are necessary to regenerate sufficient bone volume for subsequent placement of implants in the correct position and angulation. In case of advanced jaw resorption, it is often impossible to apply particulated grafting materials alone due to the absence of a natural cavity to contain the grafting material; therefore, titanium meshes in combination with bone granules or bone augmentation methods using solid bone blocks and plates are used.
Non-resorbable options: space support but with some shortcomings
Non-resorbable titanium meshes are used to create space for particulate bone grafts and provide mechanical stability for graft consolidation, especially in cases of large vertical defects. Although titanium meshes are stable and readily available, they are known for a high risk of wound dehiscence and complications. Rates of mesh exposure reported in the literature were as high as 66%.3 Consequent partial or complete removal of the exposed mesh is often necessary to prevent infection and graft resorption in the exposed area.3
Another major drawback of titanium meshes is the difficulty of achieving effective cell isolation due to its porous structure which often leads to soft tissue growth on the surface of the newly regenerated bone.3 Heavy soft tissue infiltration through the mesh also complicates the removal of the device.4 Moreover, traditional titanium meshes lack enough flexibility and are difficult to shape and trim.4 For relatively smaller defects, non-resorbable titanium membranes/foils are used. Besides the need for removal, these are also commonly associated with soft tissue complications, with reported rates of membrane exposure as high as 50%.5
The use of titanium meshes and titanium membranes can be largely avoided by relying on augmentation techniques using bone grafts that possess inherent strength such as bone blocks and cortical shells (plates). These can be obtained from the patient’s own bone (autologous graft), but they are nowadays also readily available in a processed form through donated human bone (allogeneic graft).
Autologous bone blocks: excellent biological properties at the expense of donor site morbidity and patient’s comfort
Autologous bone grafts are known for their rapid incorporation and remodeling into new bone, driven by the cells and growth factors naturally found in the graft itself; however, the need for donor sites and the complications associated with bone harvesting procedures as well as the availability of the material are still matters of concern.
Neurosensory disturbances and long-lasting paresthesia as a result of nerve irritations or partial nerve injuries are considered the most serious complications. Autologous bone grafts are commonly harvested from intraoral sites such as the mandibular symphysis (chin region) and mandibular ramus; larger volumes are harvested from the iliac crest.6 The inferior alveolar nerve (IAN) and its branches (mental nerve) and the lateral femoral cutaneous nerve (LFCN) are located in these sites and therefore are at risk of injuries and irritations.6
Temporary neurosensory disturbances of the IAN lasting up to 4 weeks postoperatively were previously reported by up to 76% and 36% of the patients following the harvesting of bone blocks from the chin region and the ramus, whereas rates of permanent sensory alterations were as high as 52% and 4%, respectively.7 Although the reported rates of permanent sensitivity changes after harvesting blocks from the ramus are relatively low, higher rates and more severe nerve injuries are expected from less experienced practitioners.
Another unfavorable outcome of harvesting autologous bone grafts is the prolonged postoperative pain (> 1 week) at the donor site which was previously reported by 33% and 20% of the patients following the harvesting of bone blocks from the chin region and ramus, respectively.7 Increased pain during chewing and increased frequency of bleeding at the donor site were specifically reported after ramus bone harvesting.8
Sensory disorders as well as pain and physical disability are also common following the harvesting of bone grafts from extraoral donor sites. Previously reported rates of local sensory changes due to injuries of the LFCN during harvesting of bone blocks from the iliac crest were as high as 52% during the first postoperative week, whereas long-term (> 12 months) sensory alterations were seen in up to 10% of the patients.9 Temporary (up to 6 weeks), prolonged (up to 4 months), and permanent pain at the donor site were previously recorded by 38%-100%, 10%-21%, and 11% of the patients, respectively.10–13
Furthermore, walking difficulties 1-5 weeks after harvesting iliac crest grafts were previously reported by 79%-100% of the patients, whereas permanent gait disturbance was present in 4% of the patients.10,12 22%-44% of the patients reported the need for walking aids such as crutches and walking sticks for up to 6.5 months.10–12 Minor and rare complications like stress fractures in the area of graft harvest, hematoma, seroma, and infection of the harvesting site were also reported.10–12 Another major disadvantage of iliac bone grafts is the need for hospitalization and sick leave which was previously reported as 4.3 days and 20.2 days on average, respectively, which is not only inconvenient for the patient but also adds costs to health services.13
The potential of autologous bone grafts is also limited by their availability. A single ramus block graft is usually harvested from only one side of the mandibula to allow the patient to have a functional side to chew on without pain and bleeding. Up to two blocks are usually harvested from the chin. Therefore, augmenting multiple defects at the same time using intraoral autologous bone is often impossible. Moreover, in case of the need for more blocks for future augmentations, the previous donor sites must completely repair and build new high-quality bone to allow for a second intervention in the same region which may take up to 2 years.14 Extraoral donor sites such as the iliac crest offer larger volumes of bone grafts; however, it was reported that the rate of complications and donor site morbidity increases with increasing size of the harvested graft.15
In order to evade the above-mentioned disadvantages of autologous bone blocks while ensuring excellent outcomes, processed allogeneic bone blocks can be used as a suitable alternative for the reconstruction of highly atrophied alveolar ridge defects.
Allogeneic bone blocks: successful alternative to autologous bone grafts
Since the late 1990s, allogeneic grafts have been used for bone regeneration in oral surgery.16 In principle, allografts function analogous to autologous bone transplants with comparable clinical results while eliminating the complications associated with bone harvesting procedures.
Since the primary goal of bone grafting procedures is to enable dental implant restoration that ideally spans decades or even a lifetime, evaluating the performance of bone blocks in terms of long-term implant survival rate (ISR) within the blocks is considered the most crucial analysis. Several studies emphasized similar ISR in either autologous or allogeneic bone blocks. The latest systematic review comparing autologous and allogeneic bone blocks with a minimum follow-up of 12 months reported mean ISRs of 96.23 ± 5.27% and 97.66 ± 2.68% for autologous and allogeneic bone blocks, respectively. The difference was not statistically significant.1 From the patient’s perspective, augmentation procedures with allogeneic blocks seemed to be advantageous, with patients reporting less pain, swelling, and sensory disorders compared to autologous block augmentations.17,18
Allogeneic bone blocks and cortical plates provide similar structural and mechanical properties as their autologous counterparts and, depending on their type and processing, they may contain the collagenous matrix and proteins of natural bone.19 Even though they lack living cells, allografts are known for their osteoconductive properties and their ability to completely remodel over time into new vital bone.20,21 Shorter surgery time and unlimited availability are another key benefits of using allografts.22 In addition, the availability of allogeneic blocks in a processed form opened the door for the opportunity to plan ahead and customize bone blocks depending on the defect geometry which facilitates the reconstruction of severe and complex defects.23
In the great scheme, allografts can be divided into two main categories: fresh frozen and processed allografts. Relatively high complication and failure rates as well as significantly lower implant success rates were previously reported for fresh frozen bone blocks compared to processed freeze-dried allografts and autologous bone blocks.1,24 Moreover, fresh frozen allografts are neither chemically processed nor gamma sterilized as in the case of processed freeze-dried allografts; therefore, all cells and tissue compounds are retained within the grafting material and the possibility of viral infections cannot be excluded completely.25 On the other hand, several studies reported similar implant success rates in freeze-dried allogeneic blocks compared to autologous bone blocks as well as good histologic findings indicating the remodeling and new building of vital bone tissue.1,21,26
Mainly two types of processed allografts are currently on the market: mineralized and demineralized allografts. Histological findings in one study showed superior osteoconductive properties of mineralized allografts where osteoblasts were found to populate the whole grafting material and the particles were surrounded by newly formed bone whereas in demineralized grafts signs of osteogenesis were only seen near the host bone.27 Nevertheless, up till now, clinical investigations have failed to reveal any significant difference between these two types of allogeneic bone grafts.28,29
Among the mineralized freeze-dried allogeneic blocks and plates currently available on the market are maxgraft® blocks, maxgraft® bonebuilder, and maxgraft® cortico. The excellent performance of these allografts in terms of implant success and gained bone volume as well as short- and long-term histological analysis has been demonstrated in several studies.
maxgraft® blocks
Cancellous maxgraft® blocks are processed from femoral heads of living donors undergoing hip replacement surgeries. These have been used for the reconstruction of three-dimensional alveolar ridge defects with clinical results comparable to autologous block augmentations.
In a retrospective study, Kloss et al. compared the performance of cancellous maxgraft® blocks to cortical autologous bone blocks harvested from the lateral aspect of the ramus and mandibular body.30 The blocks were applied as onlay grafts in a total of 42 patients (21 patients in each group) with one tooth missing and insufficient bone quantity for direct implant placement. The types of treated bone defects included non-self-containing, combined horizontal and vertical, and through-and-through defects.
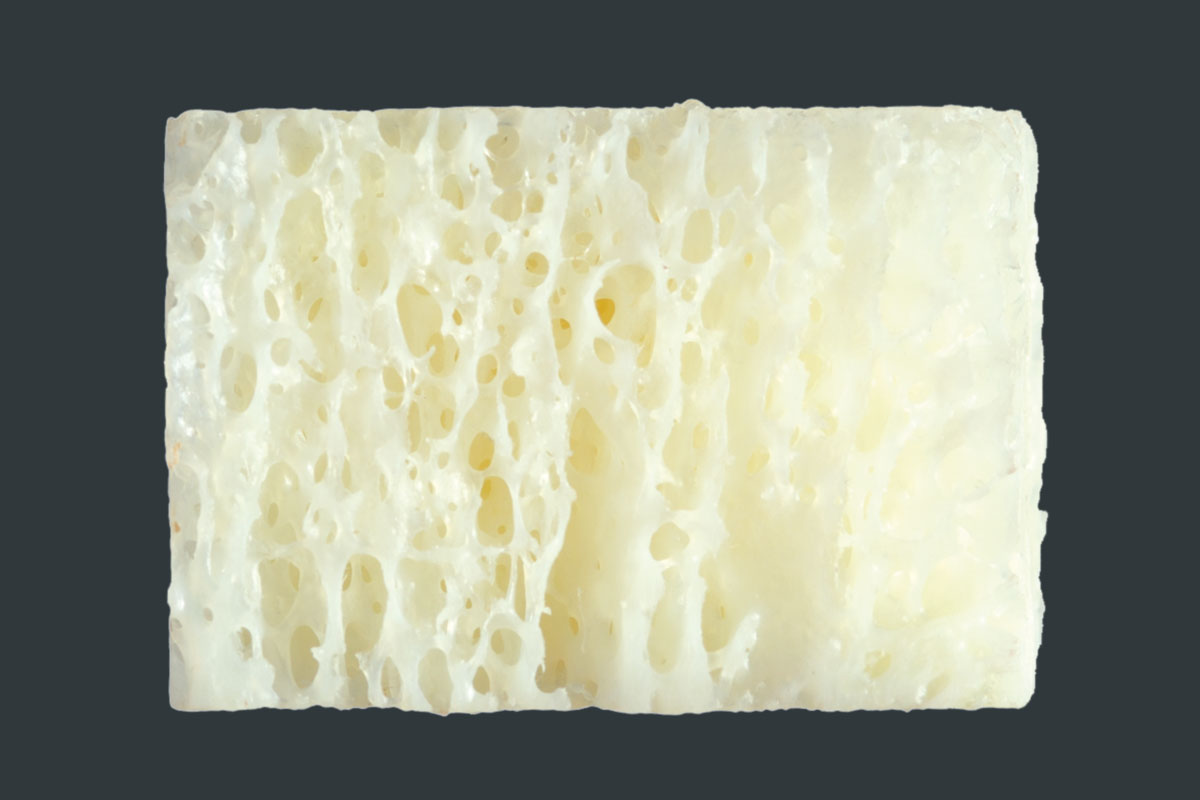
Cancellous maxgraft® block
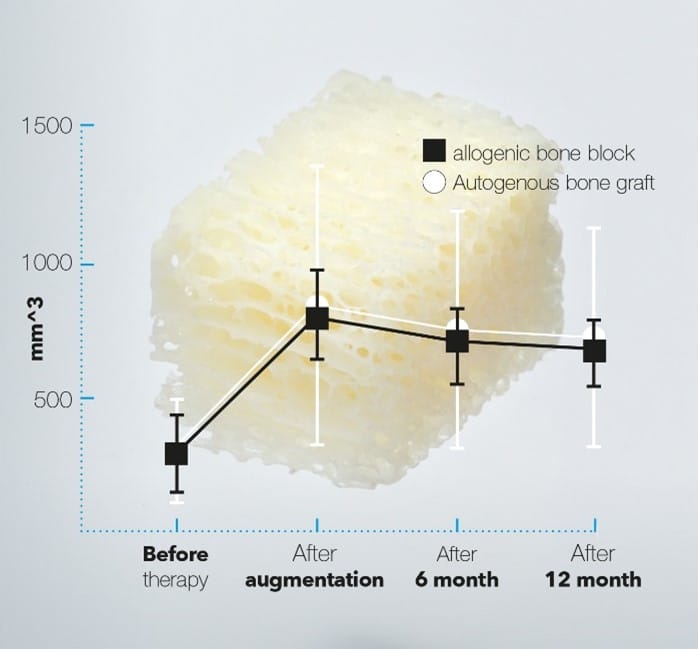
Excellent volumetric stability of allogeneic maxgraft® blocks compared with autologous cortical blocks harvested from the ramus.30
At the last follow-up of 12 months, the implant and graft success rates were 100% in both groups and there were no signs of infection, wound dehiscence, block graft exposure, or other postoperative complications.
The authors used a mathematical approach to determine the volume of the defect and to calculate the resorption/shrinkage of the bone blocks due to the remodeling process of the grafts. At 6 and 12 months no statistically significant difference in the overall volume gain was found between the autologous and maxgraft® allogeneic bone blocks. The gained volume in both groups was sufficient for implant stability and prevented the exposure of the threads. In this study, the defects were predominantly horizontal with a slight vertical component. The gain in horizontal dimensions at 12 months was similar in both groups: 5.1 mm ± 1.5 mm for autologous blocks and 5.2 mm ± 1.4 mm for allogeneic blocks.
Although the harvested autologous bone blocks were mostly cortical in nature, while the allogeneic freeze-dried maxgraft® blocks were of cancellous structure, the calculated three-dimensional shrinkage did not differ significantly between the groups: 12.5% ± 7.8% for the autologous group and 14.4% ± 9.8% for the allogeneic group. Hence, the authors concluded that excessive over-contouring is not necessary when using cancellous maxgraft® blocks.
The most common complication associated with using allogeneic bone blocks for ridge augmentation is related to the soft tissue coverage of the graft. In a recent study from 2022, Kloss et al. investigated the complications following block augmentations using maxgraft® freeze-dried allogeneic bone blocks and autologous bone blocks harvested from the lateral aspect of the ramus and mandibular body.31 Overall lower complications were observed with the allogeneic blocks (7.9%) compared to the autologous blocks (20%). The rate of wound dehiscence resulting in membrane exposure was 10% for the autologous group and 5.3% for the allogeneic group. Consequently, the reported rates of total graft failure were not higher than 2%. These rates are substantially lower than those previously reported by Chaushu et al. in 2010.32 An association between soft tissue complications and over-contouring of the blocks was reported by Kloss et al.; therefore, the relatively high complication rates reported by Chaushu et al. were assumed to be linked to the over-contouring which was a common practice at that time. The authors concluded that augmentations should stay at about the natural level of the alveolar ridge. Smoking, defect type, and poor patient compliance were also associated with more complications. The average gain in width and height was reported as 4.8 mm ± 1.2 mm and 1.1 mm ± 1.9 mm in the autologous group and 4.6 mm ± 1.6 mm and 1.3 mm ± 2.2 in the allogeneic group. The differences were not statistically different. Generally, vertical augmentations above 2.55 mm were associated with more complications regardless of the grafting material.
maxgraft® bonebuilder
Similar to the cancellous maxgraft® blocks, maxgraft® bonebuilder is a cancellous freeze-dried allogeneic bone block; however, it is customized and pre-shaped for each patient. Using the combination of CBCT scans and CAD/CAM technology, a regular bone block is milled into a shape that precisely matches the morphology of the defect.
The precise fit of the graft into the bone defect reduces empty spaces often created between the host bone and manually shaped bone blocks, thereby promoting trophic support and graft vascularization.33
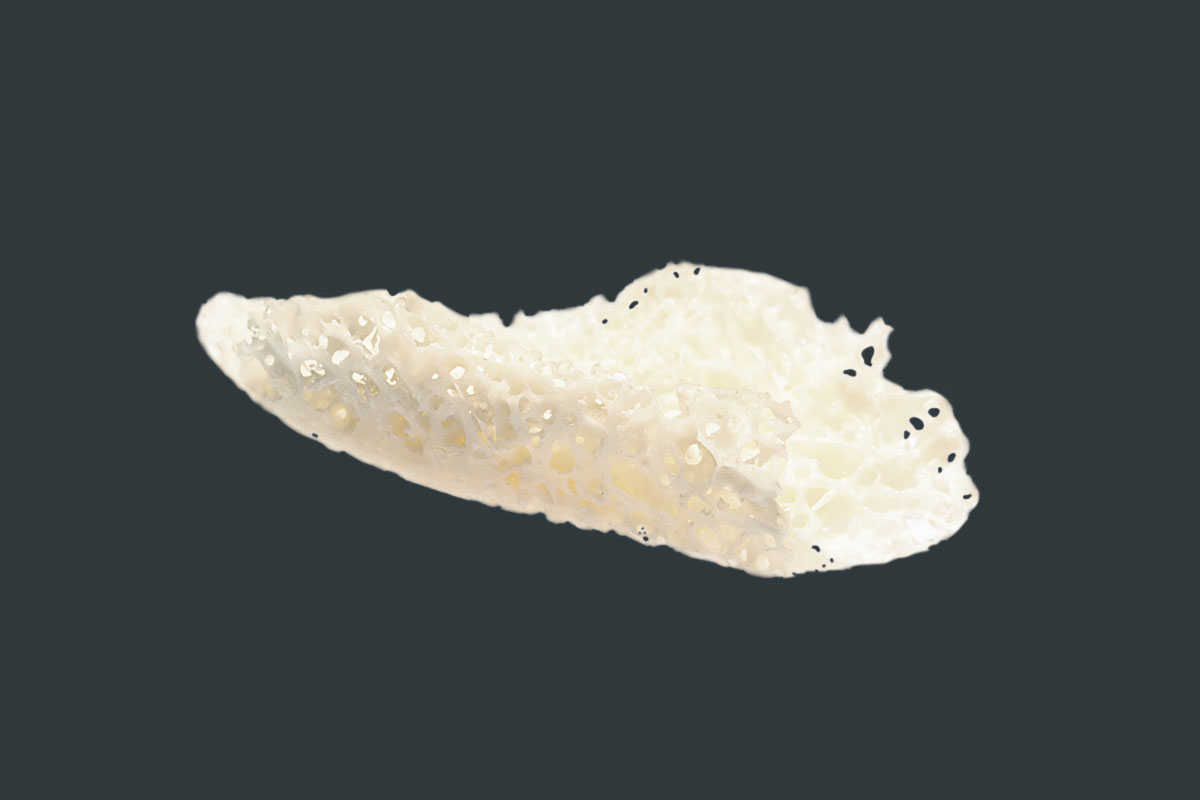
maxgraft® bonebuilder
Furthermore, the surgery time is considerably shortened using customized bone blocks because in most cases no manual adjustment of the block is required before fixation, which may also reduce the risk of contamination and the development of postoperative infections.34
Due to the opportunity for customization, maxgraft® bonebuilder is known for its versatility with reported clinical success in the mandible as well as the maxilla in posterior and anterior areas. In 2014, Jacotti et al. published a case report where maxgraft® bonebuilder was used for the reconstruction of a posterior mandible with a knife-edge configuration.35 At 7 months (time of implant insertion), 4 mm of bone was gained in the horizontal dimension, which was sufficient for proper placement of the implants.
Between the years 2017-2021, a series of cases published by the research group of Dr. Blume demonstrated the efficacy of the maxgraft® bonebuilder in the esthetic zone and the premolar area of the maxilla. 33,34,36–39 Depending on the defect size, the reported radiographic linear measurements of bone gain in the horizontal and vertical dimensions at the time of implantation were between 3.5-8 mm and 2.8-4.4 mm, respectively. In systematic reviews, similar values were reported for autologous bone blocks: 5.2 mm in the horizontal dimension and 4.12 mm in the vertical dimension.7,40
Several studies also assessed the volumetric stability of maxgraft® bonebuilder before implant placement, demonstrating limited resorption. Using volume rendering softwares, the reported resorption rates of the maxgraft® bonebuilder due to the remodeling process during the healing phase were between 3%-32%, which emphasizes the high-volume stability of the graft.23,33,37 Fluctuations in the reported data on the resorption rate of maxgraft® bonebuilder are likely attributed to the various types and severities of the treated defects as well as the differences in the sizes of the applied bone blocks. . Nevertheless, a similar resorption rate (29%) was previously reported for allogeneic cancellous freeze-dried blocks.41 Likewise, comparable rates were reported for autologous blocks harvested from the ramus and iliac crest: 27% and , respectively.42,43 Further studies with a much larger sample size are required to have a precise idea of the resorption rate of bone blocks considering the defect type, size, and location.
At the time of implant placement, the grafted area using the maxgraft® bonebuilder was described as well-integrated vascularized vital bone.34,36–38 Although the maxgraft® bonebuilder is a cancellous bone block, the formation of a cortical bone layer on the surface of the graft was observed radiographically and clinically, demonstrating the excellent regeneration potential of the material.20,34 Dental implants were inserted with torque values between 15-50 Ncm, indicating sufficient stability of the augmented sites for implant placement.34,36
of the regenerated bone at 6 months showed the complete integration of the allogeneic material in vascularized new-formed bone. The presence of multinucleated giant cells, osteoblasts, and osteoclasts around the allogeneic material indicated the ongoing remodeling process of the graft.37,38 Histomorphometric analysis showed that the biopsy specimens were composed of 41.5%-52%, 25%-29.3%, and 23%-29.2% newly formed bone, soft tissue, and residual grafting material, respectively.37,38 Long-term histological data at 5 years showed no avital remnants of the allogeneic bone block and the complete remodeling of the maxgraft® bonebuilder into new vital bone, which was indistinguishable from healthy native bone tissue.20
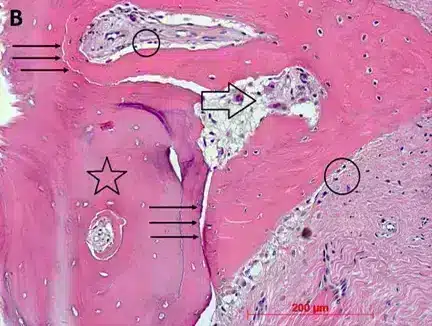
Histological analysis at 6 months shows the allogeneic material surrounded by newly formed bone (star). Osteoblasts (circle) and osteoclasts (open arrow) indicate the ongoing remodeling of the graft.33
maxgraft® cortico
maxgraft® cortico is a mineralized freeze-dried cortical plate that originates from the femoral diaphysis of post-mortem donors. It is ideal for the reconstruction of three-dimensional defects with vertical and horizontal components, especially in shallow bone defects, where it is hard to adapt regular bone blocks to the defect morphology. maxgraft® cortico is used in combination with adjusting screws to restore the contours of the alveolar ridge and simultaneously create a stable space between the shell and the local bone. The resulting container is then filled with particulated bone substitute material. This is widely known as the shell technique.
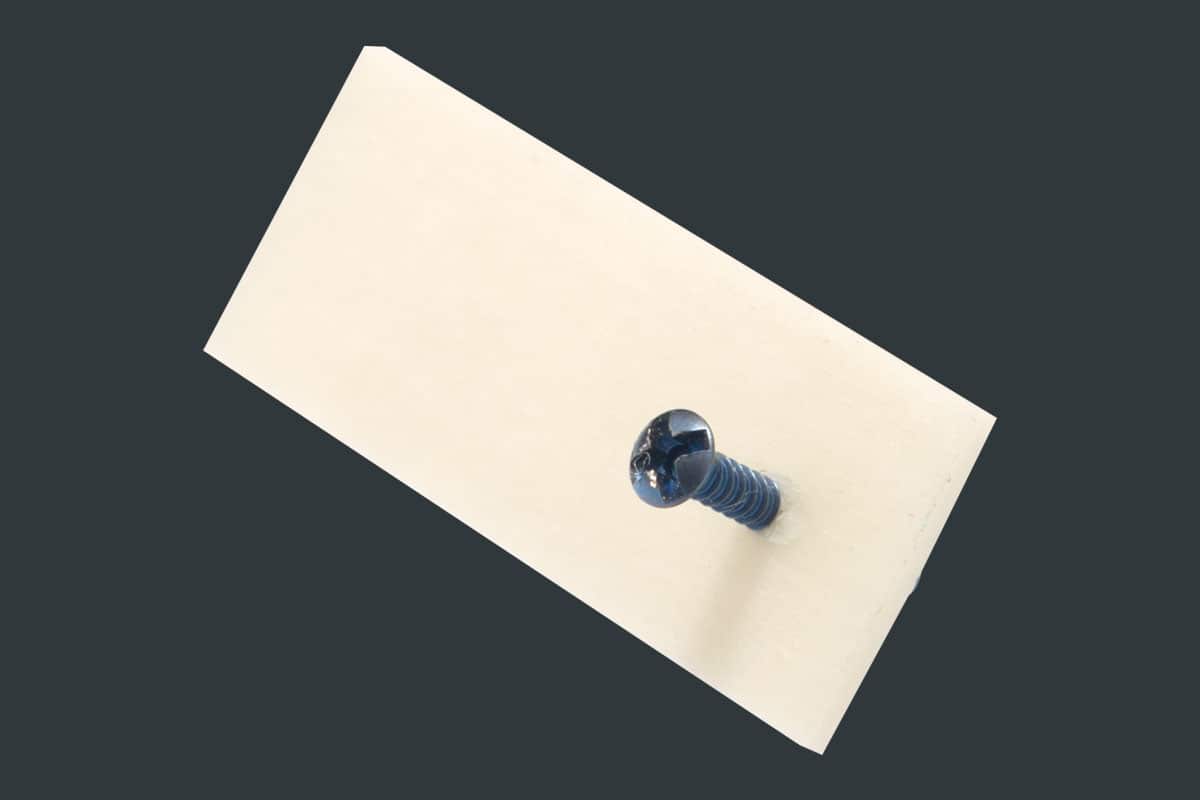
maxgraft® cortico
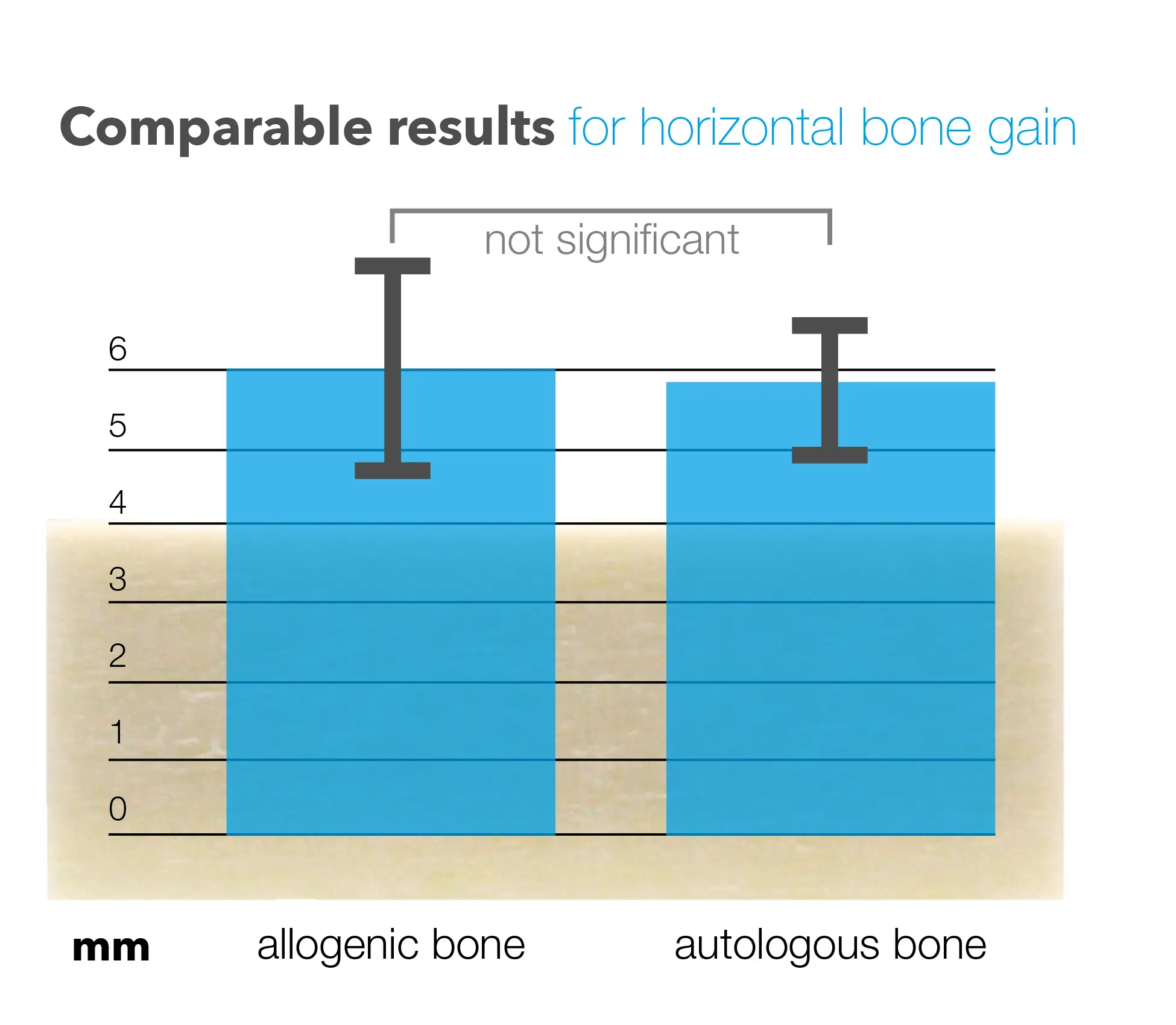
maxgraft® cortico performing similarly to autologous shells using the shell technique.44
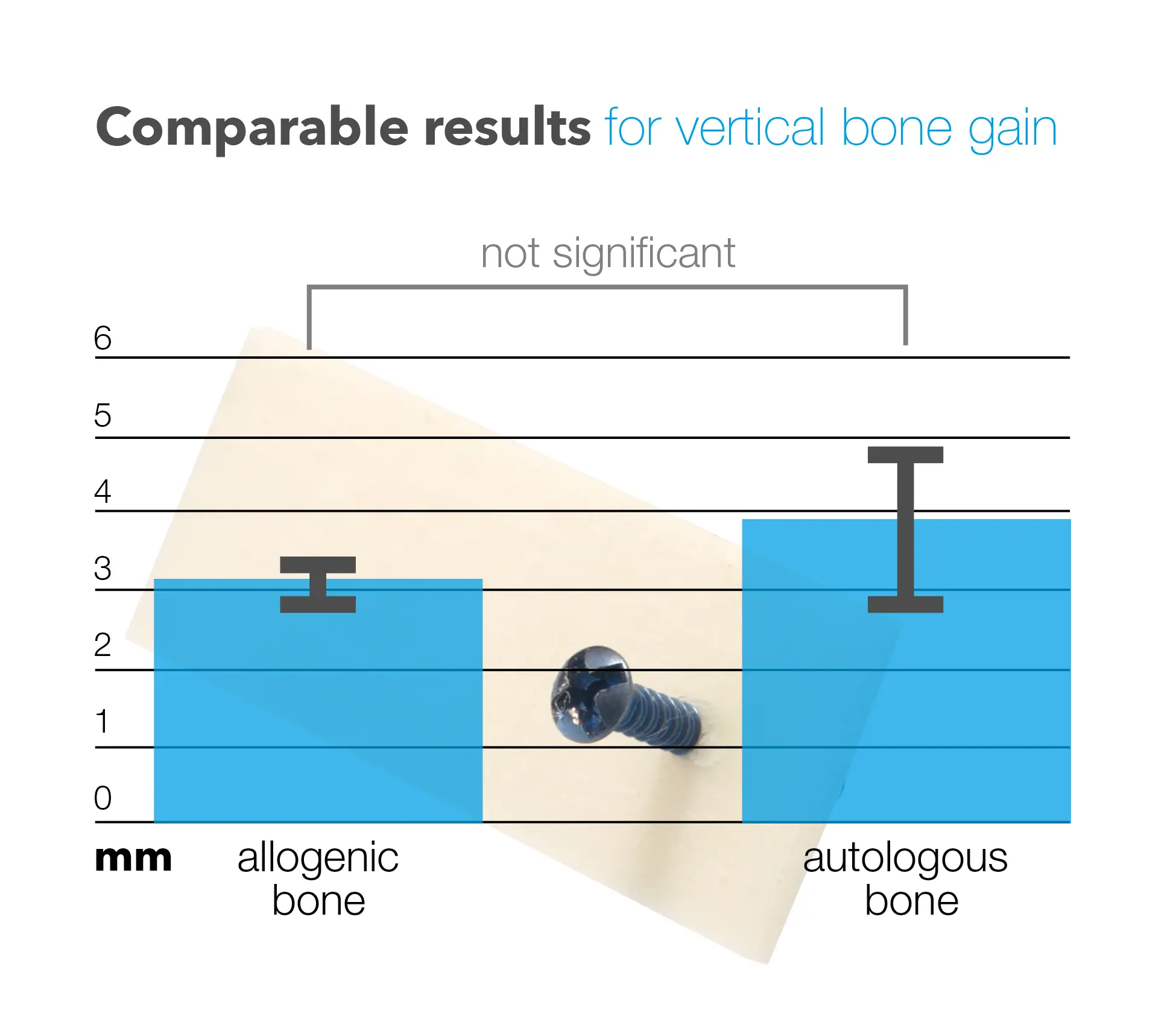
In 2020, et al. reported on five patients with bilateral atrophy of the alveolar ridge who were treated with allogeneic and autologous shells on opposite sites.44 Due to the extent of the defects, the material available from a single intraoral harvesting site was not enough to perform the augmentations. In two patients, blocks were previously harvested from the ramus on one side; therefore, a second retromolar bone harvesting procedure was not feasible. The other three patients decided to have only one bone harvesting site and chose allogeneic shells to augment the other side.
At 4-5 months (at the time of implant placement), the average horizontal gain on the autologous and allogeneic sides was 5.8 ± 0.4 mm and 6.1 ± 0.6 mm, respectively. The average vertical gain on the autologous and allogeneic sides was 3.9 ± 1.3 mm and 3.2 ± 0.3 mm, respectively. The difference between autologous and allogeneic shells in the horizontal and vertical bone gain was not statistically significant. The authors concluded that the combination of allogeneic shells with autologous bone chips is a promising alternative to autologous shells.
In a multicenter case series including 372 cases and 656 implants, et al. investigated the outcomes of different bone grafting materials for filling the container created by maxgraft® cortico.22 Space filling using allogeneic granules or a mixture of allogeneic/autologous granules demonstrated complete remodeling after 5 months; therefore these were recommended as alternatives to autologous bone granules alone. A mixture of allogeneic/bovine granules demonstrated sufficient integration and partial remodeling only within the allogeneic material, which might reduce the resorption associated with the remodeling process of the graft within the container. Complications were seen in only 8% of the cases (30 cases) which were mostly related to soft tissue management and improper fixation/positioning of the plate. In addition, the study highlighted the importance of sufficient rehydration of the plate by at least 10 minutes to prevent plate fracture.
Summary
maxgraft® blocks and plates have been shown to provide reliable solutions for reconstructing alveolar ridge defects of various types and severities, making them suitable for a broad spectrum of clinical scenarios. Their clinical success, convenience, and ability to minimize patient discomfort contribute to their popularity among dental and maxillofacial surgeons.